Table of Contents For This Article:
1. Development of Amino Acids
2. Structural properties
3. Chemical composition
4.Classification
5. Synthesis
6. Physicochemical properties
7. Toxicity
8. Antimicrobial activity
9. Rheological properties
10. Applications in the cosmetic industry
11. Applications in everyday cosmetics
Amino Acid Surfactants (AAS) are a class of surfactants formed by combining hydrophobic groups with one or more Amino Acids. In this case, the Amino Acids can be synthetic or derived from protein hydrolysates or similar renewable sources. This paper covers the details of most of the available synthetic routes for AAS and the effect of different routes on the physicochemical properties of the end products, including solubility, dispersion stability, toxicity and biodegradability. As a class of surfactants in increasing demand, the versatility of AAS due to their variable structure offers a large number of commercial opportunities.
Given that surfactants are widely used in detergents, emulsifiers, corrosion inhibitors, tertiary oil recovery and pharmaceuticals, researchers have never ceased to pay attention to surfactants.
Surfactants are the most representative chemical products that are consumed in large quantities on a daily basis around the world and have had a negative impact on the aquatic environment. Studies have shown that the widespread use of traditional surfactants can have a negative impact on the environment.
Today, non-toxicity, biodegradability and biocompatibility are almost as important to consumers as the utility and performance of surfactants.
Biosurfactants are environmentally friendly sustainable surfactants that are naturally synthesized by microorganisms such as bacteria, fungi, and yeast, or secreted extracellularly. Therefore, biosurfactants can also be prepared by molecular design to mimic natural amphiphilic structures, such as phospholipids, alkyl glycosides and acyl Amino Acids.
Amino Acid surfactants (AAS) are one of the typical surfactants, usually produced from animal or agriculturally derived raw materials. Over the past two decades, AAS have attracted a great deal of interest from scientists as novel surfactants, not only because they can be synthesized from renewable resources, but also because AAS are readily degradable and have harmless by-products, making them safer for the environment.
AAS can be defined as a class of surfactants consisting of Amino Acids containing Amino Acid groups (HO 2 C-CHR-NH 2) or Amino Acid residues (HO 2 C-CHR-NH-). The 2 functional regions of Amino Acids allow for the derivation of a wide variety of surfactants. A total of 20 standard Proteinogenic Amino Acids are known to exist in nature and are responsible for all physiological reactions in growth and life activities. They differ from each other only according to the residue R (Figure 1, pk a is the negative logarithm of the acid dissociation constant of the solution). Some are non-polar and hydrophobic, some are polar and hydrophilic, some are basic and some are acidic.
Because Amino Acids are renewable compounds, surfactants synthesized from Amino Acids also have a high potential to become sustainable and environmentally friendly. The simple and natural structure, low toxicity and rapid biodegradability often make them superior to conventional surfactants. Using renewable raw materials (e.g. Amino Acids and vegetable oils), AAS can be produced by different biotechnological routes and chemical routes.
In the early 20th century, Amino Acids were first discovered to be used as substrates for the synthesis of surfactants. AAS were mainly used as preservatives in pharmaceutical and cosmetic formulations. In addition, AAS were found to be biologically active against a variety of disease-causing bacteria, tumors, and viruses. In 1988, the availability of low-cost AAS generated research interest in surface activity. Today, with the development of biotechnology, some Amino Acids are also able to be synthesized commercially on a large scale by yeast, which indirectly proves that AAS production is more environmentally friendly.
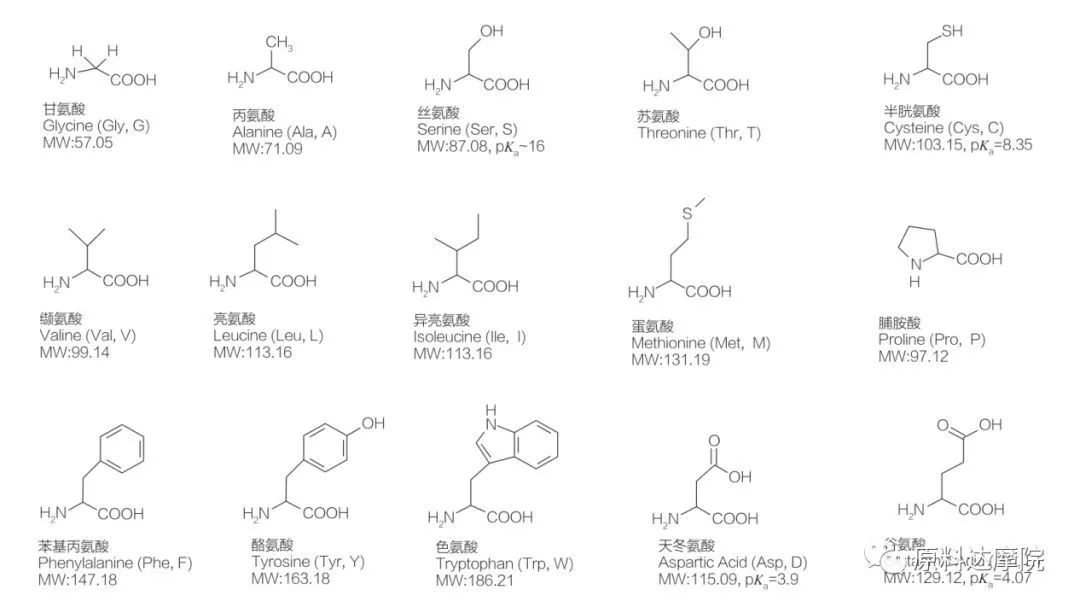
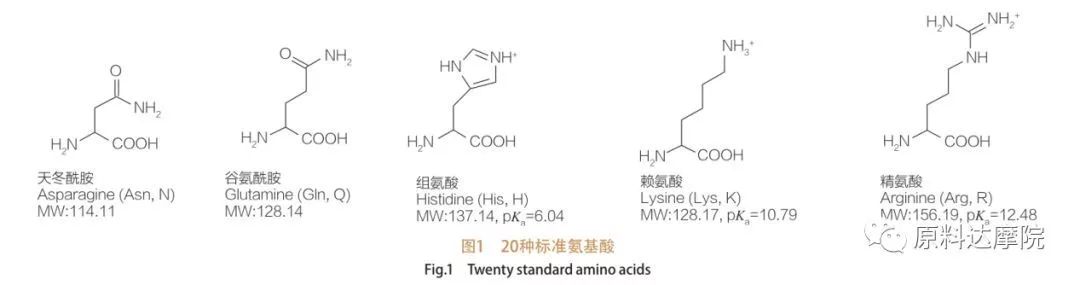
01 Development of Amino Acids
As early as the early 19th century, when naturally occurring Amino Acids were first discovered, their structures were predicted to be extremely valuable - usable as raw materials for the preparation of amphiphiles. The first study on the synthesis of AAS was reported by Bondi in 1909.
In that study, N-acylglycine and N-acylalanine were introduced as hydrophilic groups for surfactants. Subsequent work involved the synthesis of lipoAmino Acids (AAS) using glycine and alanine, and Hentrich et al. published a series of findings, including the first patent application, on the use of acyl sarcosinate and acyl aspartate salts as surfactants in household cleaning products (e.g. shampoos, detergents and toothpastes). Subsequently, many researchers investigated the synthesis and physicochemical properties of acyl Amino Acids. To date, a large body of literature has been published on the synthesis, properties, industrial applications and biodegradability of AAS.
02 Structural Properties
The non-polar hydrophobic fatty acid chains of AAS may vary in structure, chain length and number. The structural diversity and high surface activity of AAS explain their broad compositional diversity and physicochemical and biological properties. The head groups of AAS are composed of Amino Acids or peptides. The differences in the head groups determine the adsorption, aggregation and biological activity of these surfactants. The functional groups in the head group then determine the type of AAS, including cationic, anionic, nonionic, and amphoteric. The combination of hydrophilic Amino Acids and hydrophobic long-chain portions form an amphiphilic structure that makes the molecule highly surface active. In addition, the presence of asymmetric carbon atoms in the molecule helps to form chiral molecules.
03 Chemical Composition
All Peptides and Polypeptides are the Polymerization products of these nearly 20 α-Proteinogenic α-Amino Acids. All 20 α-Amino Acids contain a carboxylic acid functional group (-COOH) and an amino functional group (-NH 2), both attached to the same tetrahedral α-carbon atom. Amino Acids differ from each other by the different R groups attached to the α-carbon (except for lycine, where the R group is hydrogen.) The R groups may differ in structure, size and charge (acidity, alkalinity). These differences also determine the solubility of Amino Acids in water.
Amino Acids are chiral (except for glycine) and are optically active by nature because they have four different substituents linked to the alpha carbon. Amino Acids have two possible conformations; they are non-overlapping mirror images of each other, despite the fact that the number of L-stereoisomers is significantly higher. The R-group present in some Amino Acids (Phenylalanine, Tyrosine and Tryptophan) is aryl, leading to a maximum UV absorption at 280 nm. The acidic α-COOH and the basic α-NH 2 in Amino Acids are capable of ionization, and both stereoisomers, whichever they are, construct the ionization equilibrium shown below.
R-COOH ↔R-COO-+H+
R-NH3+↔R-NH2+H+
As shown in the ionization equilibrium above, amino acids contain at least two weakly acidic groups; however, the carboxyl group is much more acidic compared to the protonated amino group. pH 7.4, the carboxyl group is deprotonated while the amino group is protonated. Amino acids with non-ionizable R groups are electrically neutral at this pH and form zwitterion.
04 Classification
AAS can be classified according to four criteria, which are described below in turn.
4.1 According to the origin
According to the origin, AAS can be divided into 2 categories as follows. ① Natural Category
Some naturally occurring compounds containing amino acids also have the ability to reduce surface/interfacial tension, and some even exceed the efficacy of glycolipids. These AAS are also known as lipopeptides. Lipopeptides are low molecular weight compounds, usually produced by Bacillus species.
Such AAS are further divided into 3 subclasses: surfactin, iturin and fengycin.
|
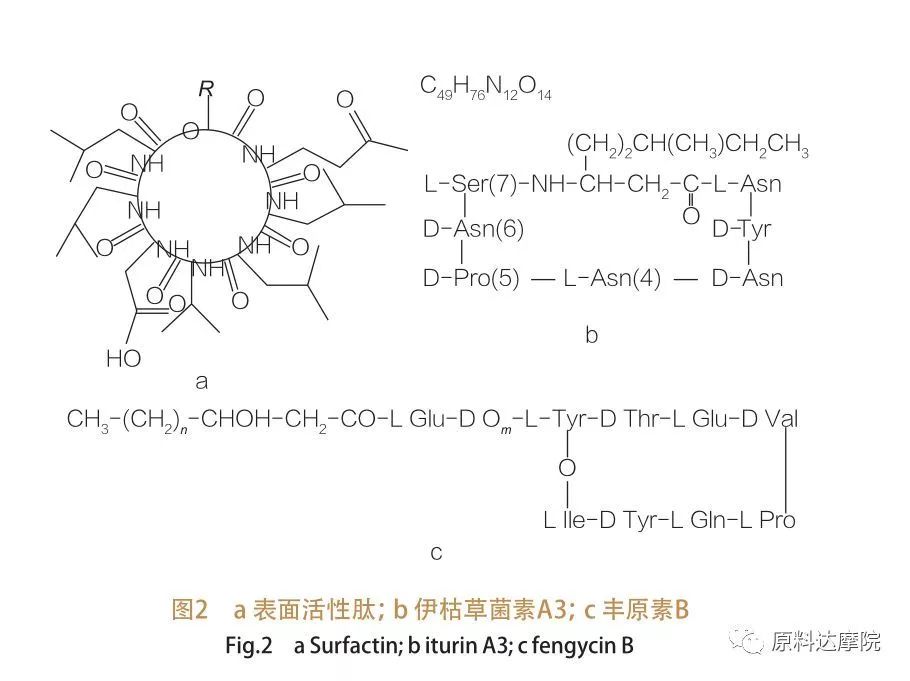
The family of surface-active peptides encompasses heptapeptide variants of a variety of substances, as shown in Figure 2a, in which a C12-C16 unsaturated β-hydroxy fatty acid chain is linked to the peptide. The surface-active peptide is a macrocyclic lactone in which the ring is closed by catalysis between the C-terminus of the β-hydroxy fatty acid and the peptide.
In the subclass of iturin, there are six main variants, namely iturin A and C, mycosubtilin and bacillomycin D, F and L. In all cases, the heptapeptides are linked to the C14-C17 chains of β-amino fatty acids (the chains can be diverse). In the case of the ekurimycins, the amino group at the β-position can form an amide bond with the C-terminus thus forming a macrocyclic lactam structure.
The subclass fengycin contains fengycin A and B, which are also called plipastatin when Tyr9 is D-configured. The decapeptide is linked to a C14 -C18 saturated or unsaturated β-hydroxy fatty acid chain. Structurally, plipastatin is also a macrocyclic lactone, containing a Tyr side chain at position 3 of the peptide sequence and forming an ester bond with the C-terminal residue, thus forming an internal ring structure (as is the case for many Pseudomonas lipopeptides).
② Synthetic Category AAS can also be synthesized by using any of the acidic, basic and neutral amino acids. Common amino acids used for the synthesis of AAS are glutamic acid, serine, proline, aspartic acid, glycine, arginine, alanine, leucine, and protein hydrolysates. This subclass of surfactants can be prepared by chemical, enzymatic, and chemoenzymatic methods; however, for the production of AAS, chemical synthesis is more economically feasible. Common examples include N-lauroyl-L-glutamic acid and N-palmitoyl-L-glutamic acid.
|
4.2 Based on aliphatic chain substituents
Based on the aliphatic chain substituents, amino acid-based surfactants can be divided into 2 types.
According to the position of the substituent
①N-substituted AAS
In N-substituted compounds, an amino group is replaced by a lipophilic group or a carboxyl group, resulting in a loss of basicity. the simplest example of N-substituted AAS are N-acyl amino acids, which are essentially anionic surfactants. n-substituted AAS have an amide bond attached between the hydrophobic and hydrophilic portions. The amide bond has the ability to form a hydrogen bond, which facilitates the degradation of this surfactant in an acidic environment, thus making it biodegradable.
②C-substituted AAS In C-substituted compounds, the substitution occurs at the carboxyl group (via an amide or ester bond). Typical C-substituted compounds (e.g. esters or amides) are essentially cationic surfactants.
③N- and C-substituted AAS In this type of surfactant, both the amino and carboxyl groups are the hydrophilic part. This type is essentially an amphoteric surfactant. |
4.3 According to the number of hydrophobic tails
Based on the number of head groups and hydrophobic tails, AAS can be divided into four groups. Straight-chain AAS, Gemini (dimer) type AAS, Glycerolipid type AAS, and bicephalic amphiphilic (Bola) type AAS. straight-chain surfactants are surfactants consisting of amino acids with only one hydrophobic tail (Figure 3). Gemini type AAS have two amino acid polar head groups and two hydrophobic tails per molecule (Figure 4). In this type of structure, the two straight-chain AAS are linked together by a spacer and are therefore also called dimers. In the Glycerolipid type AAS, on the other hand, the two hydrophobic tails are attached to the same amino acid head group. These surfactants can be considered as analogs of monoglycerides, diglycerides and phospholipids, while in Bola-type AAS, two amino acid head groups are linked by a hydrophobic tail.
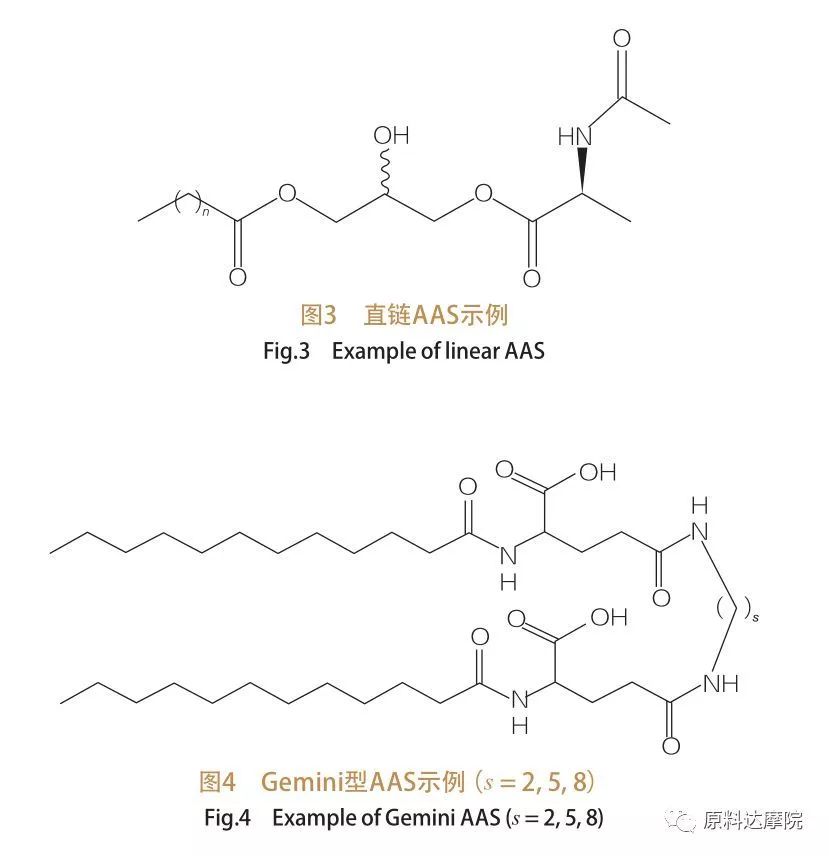
4.4 According to the type of head group
①Cationic AAS
The head group of this type of surfactant has a positive charge. The earliest cationic AAS is ethyl cocoyl arginate, which is a pyrrolidone carboxylate. The unique and diverse properties of this surfactant make it useful in disinfectants, antimicrobial agents, antistatic agents, hair conditioners, as well as being gentle on the eyes and skin and readily biodegradable. Singare and Mhatre synthesized arginine-based cationic AAS and evaluated their physicochemical properties. In this study, they claimed high yields of the products obtained using Schotten-Baumann reaction conditions. With increasing alkyl chain length and hydrophobicity, the surface activity of the surfactant was found to increase and the Critical Micelle Concentration (cmc) to decrease. Another one is the quaternary acyl protein, which is commonly used as a conditioner in hair care products.
②Anionic AAS
In anionic surfactants, the polar head group of the surfactant has a negative charge. Sarcosine (CH 3 -NH-CH 2 -COOH, N-methylglycine), an amino acid commonly found in sea urchins and sea stars, is chemically related to glycine (NH 2 -CH 2 -COOH,), a basic amino acid found in mammalian cells. -COOH,) is chemically related to glycine, which is a basic amino acid found in mammalian cells. Lauric acid, tetradecanoic acid, oleic acid and their halides and esters are commonly used to synthesize sarcosinate surfactants. Sarcosinates are inherently mild and are therefore commonly used in mouthwashes, shampoos, spray shaving foams, sunscreens, skin cleansers, and other cosmetic products.
Other commercially available anionic AAS include Amisoft CS-22 and AmiliteGCK-12, which are trade names for sodium N-cocoyl-L-glutamate and potassium N-cocoyl glycinate, respectively. Amilite is commonly used as a foaming agent, detergent, solubilizer, emulsifier and dispersant, and has many applications in cosmetics, such as shampoos, bath soaps, body washes, toothpastes, facial cleansers, cleansing soaps, contact lens cleaners and household surfactants. Amisoft is used as a mild skin and hair cleanser, mainly in facial and body cleansers, block synthetic detergents, body care products, shampoos and other skin care products.
③zwitterionic or amphoteric AAS
Amphoteric surfactants contain both acidic and basic sites and can therefore change their charge by changing the pH value. In alkaline media they behave like anionic surfactants, while in acidic environments they behave like cationic surfactants and in neutral media like amphoteric surfactants. Lauryl lysine (LL) and alkoxy (2-hydroxypropyl) arginine are the only known amphoteric surfactants based on amino acids. LL is a condensation product of lysine and lauric acid. Due to its amphoteric structure, LL is insoluble in almost all types of solvents, except for very alkaline or acidic solvents. As an organic powder, LL has excellent adhesion to hydrophilic surfaces and a low coefficient of friction, giving this surfactant excellent lubricating ability. LL is widely used in skin creams and hair conditioners, and is also used as a lubricant.
④Nonionic AAS
Nonionic surfactants are characterized by polar head groups without formal charges. eight new ethoxylated nonionic surfactants were prepared by Al-Sabagh et al. from oil-soluble α-amino acids. In this process, L-phenylalanine (LEP) and L-leucine were first esterified with hexadecanol, followed by amidation with palmitic acid to give two amides and two esters of α-amino acids. The amides and esters then underwent condensation reactions with ethylene oxide to prepare three phenylalanine derivatives with different numbers of polyoxyethylene units (40, 60 and 100). These nonionic AAS were found to have good detergency and foaming properties.
05 Synthesis
5.1 Basic synthetic route
In AAS, hydrophobic groups can be attached to amine or carboxylic acid sites, or through the side chains of amino acids. Based on this, four basic synthetic routes are available, as shown in Figure 5.
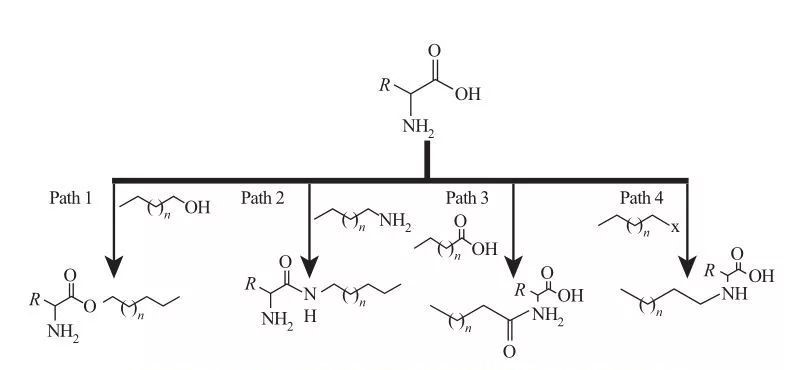
Fig.5 Fundamental synthesis paths of amino acid-based surfactants
Pathway 1.
Amphiphilic ester amines are produced by esterification reactions, in which case the surfactant synthesis is usually achieved by refluxing fatty alcohols and amino acids in the presence of a dehydrating agent and an acidic catalyst. In some reactions, sulfuric acid acts as both a catalyst and a dehydrating agent.
Pathway 2. Activated amino acids react with alkylamines to form amide bonds, resulting in the synthesis of amphiphilic amidoamines.
Pathway 3. Amido acids are synthesized by reacting the amine groups of amino acids with Amido Acids.
Pathway 4. Long-chain alkyl amino acids were synthesized by the reaction of amine groups with haloalkanes. |
5.2 Advances in synthesis and production
5.2.1 Synthesis of single-chain amino acid/peptide surfactants
N-acyl or O-acyl amino acids or peptides can be synthesized by enzyme-catalyzed acylation of amine or hydroxyl groups with fatty acids. The earliest report on the solvent-free lipase-catalyzed synthesis of amino acid amide or methyl ester derivatives used Candida antarctica, with yields ranging from 25% to 90% depending on the target amino acid. Methyl ethyl ketone has also been used as a solvent in some reactions. Vonderhagen et al. also described lipase and protease-catalyzed N-acylation reactions of amino acids, protein hydrolysates and/or their derivatives using a mixture of water and organic solvents (e.g., dimethylformamide/water) and methyl butyl ketone.
In the early days, the main problem with enzyme-catalyzed synthesis of AAS was the low yields. According to Valivety et al. the yield of N-tetradecanoyl amino acid derivatives was only 2%-10% even after using different lipases and incubating at 70°C for many days. Montet et al. also encountered problems concerning the low yield of amino acids in the synthesis of N-acyl lysine using fatty acids and vegetable oils. According to them, the maximum yield of the product was 19% under solvent-free conditions and using organic solvents. the same problem was encountered by Valivety et al. in the synthesis of N-Cbz-L-lysine or N-Cbz-lysine methyl ester derivatives.
In this study, they claimed that the yield of 3-O-tetradecanoyl-L-serine was 80% when using N-protected serine as a substrate and Novozyme 435 as a catalyst in a molten solvent-free environment. Nagao and Kito studied the O-acylation of L-serine, L-homoserine, L-threonine and L-tyrosine (LET) when using lipase The results of the reaction (lipase was obtained by Candida cylindracea and Rhizopus delemar in aqueous buffer medium) and reported that the yields of acylation of L-homoserine and L-serine were somewhat low, while no acylation of L-threonine and LET occurred.
Many researchers have supported the use of inexpensive and readily available substrates for the synthesis of cost-effective AAS. Soo et al. claimed that the preparation of palm oil-based surfactants works best with immobilized lipoenzyme. They noted that the yield of the products would be better despite the time consuming reaction (6 days). Gerova et al. investigated the synthesis and surface activity of chiral N-palmitoyl AAS based on methionine, proline, leucine, threonine, phenylalanine and phenylglycine in a cyclic/racemic mixture. Pang and Chu described the synthesis of amino acid based monomers and dicarboxylic acid based monomers in solution A series of functional and biodegradable amino acid-based polyamide esters were synthesized by co-condensation reactions in solution.
Cantaeuzene and Guerreiro reported the esterification of carboxylic acid groups of Boc-Ala-OH and Boc-Asp-OH with long-chain aliphatic alcohols and diols, with dichloromethane as solvent and agarose 4B (Sepharose 4B) as catalyst. In this study, the reaction of Boc-Ala-OH with fatty alcohols up to 16 carbons gave good yields (51%), while for Boc-Asp-OH 6 and 12 carbons were better, with a corresponding yield of 63% [64]. 99.9%) in yields ranging from 58% to 76%, which were synthesized by the formation of amide bonds with various long-chain alkylamines or ester bonds with fatty alcohols by Cbz-Arg-OMe, where papain acted as a catalyst.
5.2.2 Synthesis of gemini-based amino acid/peptide surfactants
Amino acid-based gemini surfactants consist of two straight-chain AAS molecules linked head-to-head to each other by a spacer group. There are 2 possible schemes for the chemoenzymatic synthesis of gemini-type amino acid-based surfactants (Figures 6 and 7). In Figure 6, 2 amino acid derivatives are reacted with the compound as a spacer group and then 2 hydrophobic groups are introduced. In Figure 7, the 2 straight-chain structures are directly linked together by a bifunctional spacer group.
The earliest development of enzyme-catalyzed synthesis of gemini lipoamino acids was pioneered by Valivety et al. Yoshimura et al. investigated the synthesis, adsorption and aggregation of an amino acid-based gemini surfactant based on cystine and n-alkyl bromide. The synthesized surfactants were compared with the corresponding monomeric surfactants. Faustino et al. described the synthesis of anionic urea-based monomeric AAS based on L-cystine, D-cystine, DL-cystine, L-cysteine, L-methionine and L-sulfoalanine and their pairs of gemini by means of conductivity, equilibrium surface tension and steady-state fluorescence characterization of them. It was shown that the cmc value of gemini was lower by comparing monomer and gemini.
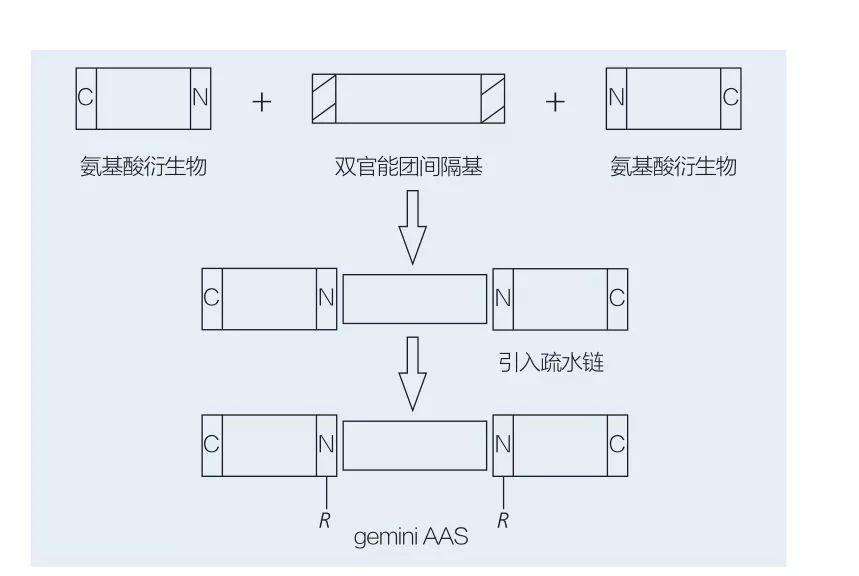
Fig.6 Synthesis of gemini AAS using AA derivatives and spacer, followed by insertion of the hydrophobic group
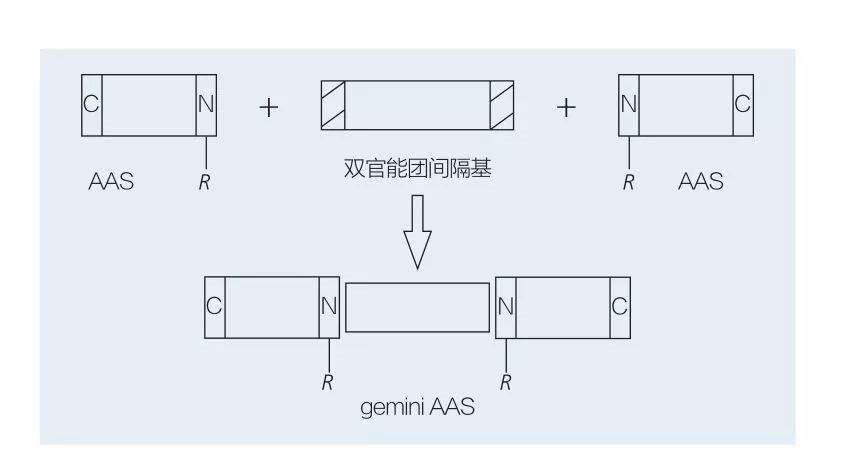
Fig.7 Synthesis of gemini AASs using bifunctional spacer and AAS
5.2.3 Synthesis of glycerolipid amino acid/peptide surfactants
Glycerolipid amino acid/peptide surfactants are a new class of lipid amino acids that are structural analogs of glycerol mono- (or di-) esters and phospholipids, due to their structure of one or two fatty chains with one amino acid linked to the glycerol backbone by an ester bond. The synthesis of these surfactants starts with the preparation of glycerol esters of amino acids at elevated temperatures and in the presence of an acidic catalyst (e.g. BF 3). Enzyme-catalyzed synthesis (using hydrolases, proteases and lipases as catalysts) is also a good option (Figure 8).
The enzyme-catalyzed synthesis of dilaurylated arginine glycerides conjugates using papain has been reported. Synthesis of diacylglycerol ester conjugates from acetylarginine and evaluation of their physicochemical properties have also been reported.
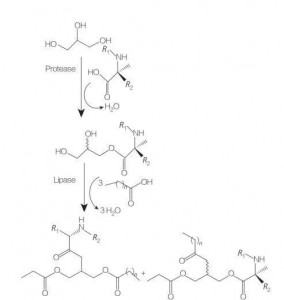
Fig.8 Synthesis of mono and diacylglycerol amino acid conjugates
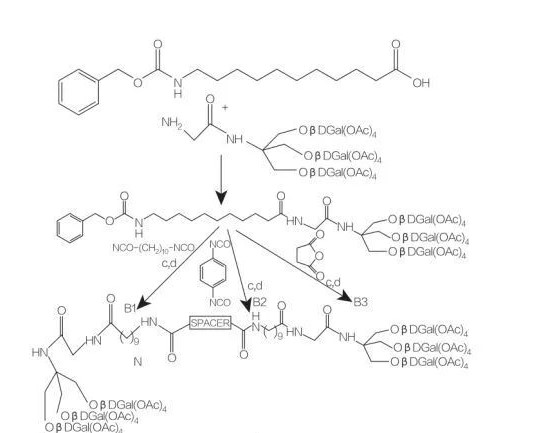
spacer: NH-(CH2)10-NH: compoundB1
spacer: NH-C6H4-NH: compoundB2
spacer: CH2-CH2: compoundB3
Fig.9 Synthesis of symmetric amphiphiles derived from Tris(hydroxymethyl)aminomethane
5.2.4 Synthesis of bola-based amino acid/peptide surfactants
Amino acid-based bola-type amphiphiles contain 2 amino acids that are linked to the same hydrophobic chain. Franceschi et al. described the synthesis of bola-type amphiphiles with 2 amino acids (D- or L-alanine or L-histidine) and 1 alkyl chain of different lengths and investigated their surface activity. They discuss the synthesis and aggregation of novel bola-type amphiphiles with an amino acid fraction (using either an uncommon β-amino acid or an alcohol) and a C12 -C20 spacer group. The uncommon β-amino acids used can be a sugar aminoacid, an azidothymin (AZT)-derived amino acid, a norbornene amino acid, and an amino alcohol derived from AZT (Figure 9). the synthesis of symmetrical bola-type amphiphiles derived from tris(hydroxymethyl)aminomethane (Tris) (Figure 9).
06 Physicochemical properties
It is well known that amino acid based surfactants (AAS) are diverse and versatile in nature and have good applicability in many applications such as good solubilization, good emulsification properties, high efficiency, high surface activity performance and good resistance to hard water (calcium ion tolerance).
Based on the surfactant properties of amino acids (e.g. surface tension, cmc, phase behavior and Krafft temperature), the following conclusions were reached after extensive studies - the surface activity of AAS is superior to that of its conventional surfactant counterpart.
6.1 Critical Micelle Concentration (cmc)
Critical micelle concentration is one of the important parameters of surfactants and governs many surface active properties such as solubilization, cell lysis and its interaction with biofilms, etc. In general, increasing the chain length of the hydrocarbon tail (increasing hydrophobicity) leads to a decrease in the cmc value of the surfactant solution, thus increasing its surface activity. Surfactants based on amino acids usually have lower cmc values compared to conventional surfactants.
Through different combinations of head groups and hydrophobic tails (mono-cationic amide, bi-cationic amide, bi-cationic amide-based ester), Infante et al. synthesized three arginine-based AAS and studied their cmc and γcmc (surface tension at cmc), showing that the cmc and γcmc values decreased with increasing hydrophobic tail length. In another study, Singare and Mhatre found that the cmc of N-α-acylarginine surfactants decreased with increasing the number of hydrophobic tail carbon atoms (Table 1).
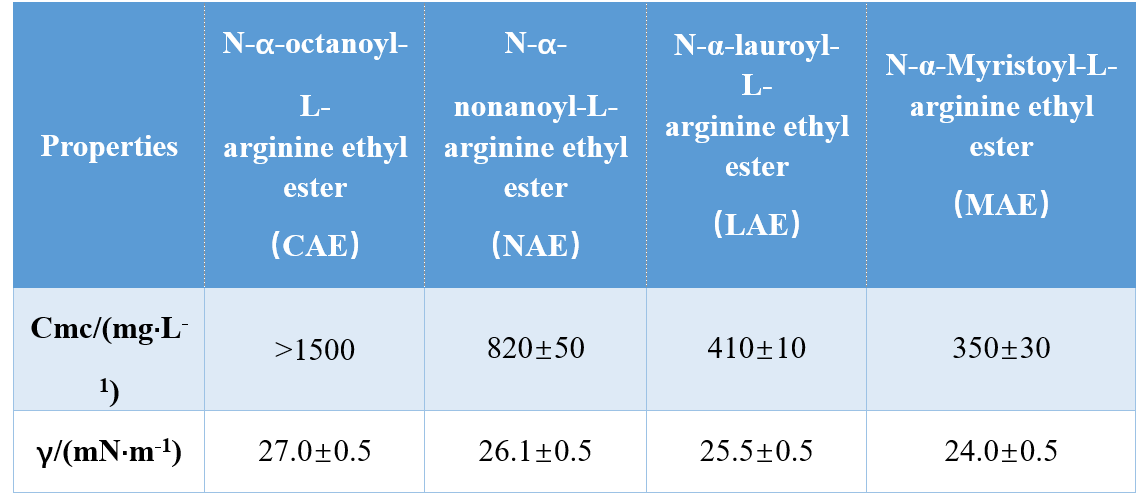
Yoshimura et al. investigated the cmc of cysteine-derived amino acid-based gemini surfactants and showed that the cmc decreased when the carbon chain length in the hydrophobic chain was increased from 10 to 12. Further increasing the carbon chain length to 14 resulted in an increase in cmc, which confirmed that long-chain gemini surfactants have a lower tendency to aggregate.
Faustino et al. reported the formation of mixed micelles in aqueous solutions of anionic gemini surfactants based on cystine. The gemini surfactants were also compared with the corresponding conventional monomeric surfactants (C 8 Cys). The cmc values of lipid-surfactant mixtures were reported to be lower than those of pure surfactants. gemini surfactants and 1,2-diheptanoyl-sn-glyceryl-3-phosphocholine, a water-soluble, micelle-forming phospholipid, had cmc in the millimolar level.
Shrestha and Aramaki investigated the formation of viscoelastic worm-like micelles in aqueous solutions of mixed amino acid-based anionic-nonionic surfactants in the absence of admixture salts. In this study, N-dodecyl glutamate was found to have a higher Krafft temperature; however, when neutralized with the basic amino acid L-lysine, it generated micelles and the solution started behaving like a Newtonian fluid at 25 °C.
6.2 Good water solubility
The good water solubility of AAS is due to the presence of additional CO-NH bonds. This makes AAS more biodegradable and environmentally friendly than the corresponding conventional surfactants. The water solubility of N-acyl-L-glutamic acid is even better due to its 2 carboxyl groups. The water solubility of Cn(CA) 2 is also good because there are 2 ionic arginine groups in 1 molecule, which results in more effective adsorption and diffusion at the cell interface and even effective bacterial inhibition at lower concentrations.
6.3 Krafft temperature and Krafft point
Krafft temperature can be understood as the specific solubility behavior of surfactants whose solubility increases sharply above a particular temperature. Ionic surfactants have a tendency to generate solid hydrates, which can precipitate out of water. At a particular temperature (the so-called Krafft temperature), a dramatic and discontinuous increase in the solubility of surfactants is usually observed. The Krafft point of an ionic surfactant is its Krafft temperature at cmc.
This solubility characteristic is usually seen for ionic surfactants and can be explained as follows: the solubility of the surfactant free monomer is limited below the Krafft temperature until the Krafft point is reached, where its solubility gradually increases due to micelle formation. To ensure complete solubility, it is necessary to prepare surfactant formulations at temperatures above the Krafft point.
The Krafft temperature of AAS has been studied and compared with that of conventional synthetic surfactants.Shrestha and Aramaki studied the Krafft temperature of arginine-based AAS and found that the critical micelle concentration exhibited aggregation behavior in the form of pre-micelles above 2-5×10-6 mol-L -1 followed by normal micelle formation ( Ohta et al. synthesized six different types of N-hexadecanoyl AAS and discussed the relationship between their Krafft temperature and amino acid residues.
In the experiments, it was found that the Krafft temperature of N-hexadecanoyl AAS increased with decreasing size of amino acid residues (phenylalanine being an exception), while the heat of solubility (heat uptake) increased with decreasing size of amino acid residues (with the exception of glycine and phenylalanine). It was concluded that in both alanine and phenylalanine systems, the D-L interaction is stronger than the L-L interaction in the solid form of the N-hexadecanoyl AAS salt.
Brito et al. determined the Krafft temperature of three series of novel amino acid-based surfactants using differential scanning microcalorimetry and found that changing the trifluoroacetate ion to iodide ion resulted in a significant increase in Krafft temperature (about 6 °C), from 47 °C to 53 °C. The presence of cis-double bonds and the unsaturation present in the long-chain Ser-derivatives led to a significant decrease in the Krafft temperature. n-Dodecyl glutamate was reported to have a higher Krafft temperature. However, neutralization with the basic amino acid L-lysine resulted in the formation of micelles in solution that behaved like Newtonian fluids at 25 °C.
6.4 Surface tension
The surface tension of surfactants is related to the chain length of the hydrophobic part. Zhang et al. determined the surface tension of sodium cocoyl glycinate by Wilhelmy plate method (25±0.2)°C and determined the surface tension value at cmc as 33 mN-m -1 , cmc as 0.21 mmol-L -1. Yoshimura et al. determined the surface tension of 2C n Cys type amino acid based surface surface tension of 2C n Cys-based surface active agents. It was found that the surface tension at cmc decreased with increasing chain length (until n = 8), while the trend was reversed for surfactants with n = 12 or longer chain lengths.
The effect of CaC1 2 on the surface tension of dicarboxylated amino acid-based surfactants has also been studied. In these studies, CaC1 2 was added to aqueous solutions of three dicarboxylated amino acid-type surfactants (C12 MalNa 2, C12 AspNa 2, and C12 GluNa 2). The plateau values after cmc were compared and it was found that the surface tension decreased at very low CaC1 2 concentrations. This is due to the effect of calcium ions on the arrangement of the surfactant at the gas-water interface. the surface tensions of the salts of N-dodecylaminomalonate and N-dodecylaspartate, on the other hand, were also almost constant up to 10 mmol-L -1 CaC1 2 concentration. Above 10 mmol-L -1, the surface tension increases sharply, due to the formation of a precipitation of the calcium salt of the surfactant. For the disodium salt of N-dodecyl glutamate, moderate addition of CaC1 2 resulted in a significant decrease in surface tension, while continued increase in CaC1 2 concentration no longer caused significant changes.
To determine the adsorption kinetics of gemini-type AAS at the gas-water interface, the dynamic surface tension was determined using the maximum bubble pressure method. The results showed that for the longest test time, the 2C 12 Cys dynamic surface tension did not change. The decrease of the dynamic surface tension depends only on the concentration, the length of the hydrophobic tails, and the number of hydrophobic tails. Increasing concentration of surfactant, decreasing chain length as well as the number of chains resulted in a more rapid decay. The results obtained for higher concentrations of C n Cys (n = 8 to 12) were found to be very close to the γ cmc measured by the Wilhelmy method.
In another study, the dynamic surface tensions of sodium dilauryl cystine (SDLC) and sodium didecamino cystine were determined by the Wilhelmy plate method, and in addition, the equilibrium surface tensions of their aqueous solutions were determined by the drop volume method. The reaction of disulfide bonds was further investigated by other methods as well. The addition of mercaptoethanol to 0.1 mmol-L -1SDLC solution led to a rapid increase in surface tension from 34 mN-m -1 to 53 mN-m -1. Since NaClO can oxidize the disulfide bonds of SDLC to sulfonic acid groups, no aggregates were observed when NaClO (5 mmol-L -1 ) was added to the 0.1 mmol-L -1 SDLC solution. Transmission electron microscopy and dynamic light scattering results showed that no aggregates were formed in the solution. The surface tension of SDLC was found to increase from 34 mN-m -1 to 60 mN-m -1 over a period of 20 min.
6.5 Binary surface interactions
In the life sciences, a number of groups have studied the vibrational properties of mixtures of cationic AAS (diacylglycerol arginine-based surfactants) and phospholipids at the gas-water interface, finally concluding that this non-ideal property causes the prevalence of electrostatic interactions.
6.6 Aggregation properties
Dynamic light scattering is commonly used to determine the aggregation properties of amino acid-based monomers and gemini surfactants at concentrations above cmc, yielding an apparent hydrodynamic diameter D H (= 2R H ). The aggregates formed by C n Cys and 2Cn Cys are relatively large and have a wide scale distribution compared to other surfactants. All surfactants except 2C 12 Cys typically form aggregates of about 10 nm. micelle sizes of gemini surfactants are significantly larger than those of their monomeric counterparts. An increase in hydrocarbon chain length also leads to an increase in micelle size. ohta et al. described the aggregation properties of three different stereoisomers of N-dodecyl-phenyl-alanyl-phenyl-alanine tetramethylammonium in aqueous solution and showed that the diastereoisomers have the same critical aggregation concentration in aqueous solution. Iwahashi et al. investigated by circular dichroism, NMR and vapor pressure osmometry the The formation of chiral aggregates of N-dodecanoyl-L-glutamic acid, N-dodecanoyl-L-valine and their methyl esters in different solvents (such as tetrahydrofuran, acetonitrile, 1,4-dioxane and 1,2-dichloroethane) with rotational properties was investigated by circular dichroism, NMR and vapor pressure osmometry.
6.7 Interfacial adsorption
The interfacial adsorption of amino acid-based surfactants and its comparison with its conventional counterpart is also one of the research directions. For example, the interfacial adsorption properties of dodecyl esters of aromatic amino acids obtained from LET and LEP were investigated. The results showed that LET and LEP exhibited lower interfacial areas at the gas-liquid interface and at the water/hexane interface, respectively.
Bordes et al. investigated the solution behavior and adsorption at the gas-water interface of three dicarboxylated amino acid surfactants, the disodium salts of dodecyl glutamate, dodecyl aspartate, and aminomalonate (with 3, 2, and 1 carbon atoms between the two carboxyl groups, respectively). According to this report, the cmc of the dicarboxylated surfactants was 4-5 times higher than that of the monocarboxylated dodecyl glycine salt. This is attributed to the formation of hydrogen bonds between the dicarboxylated surfactants and neighboring molecules through the amide groups therein.
6.8 Phase behavior
Isotropic discontinuous cubic phases are observed for surfactants at very high concentrations. Surfactant molecules with very large head groups tend to form aggregates of smaller positive curvature. marques et al. studied the phase behavior of the 12Lys12/12Ser and 8Lys8/16Ser systems (see Figure 10), and the results showed that the 12Lys12/12Ser system has a phase separation zone between the micellar and vesicular solution regions, while the 8Lys8/16Ser system The 8Lys8/16Ser system shows a continuous transition (elongated micellar phase region between the small micellar phase region and the vesicle phase region). It should be noted that for the vesicle region of the 12Lys12/12Ser system, vesicles are always coexisting with micelles, while the vesicle region of the 8Lys8/16Ser system has only vesicles.
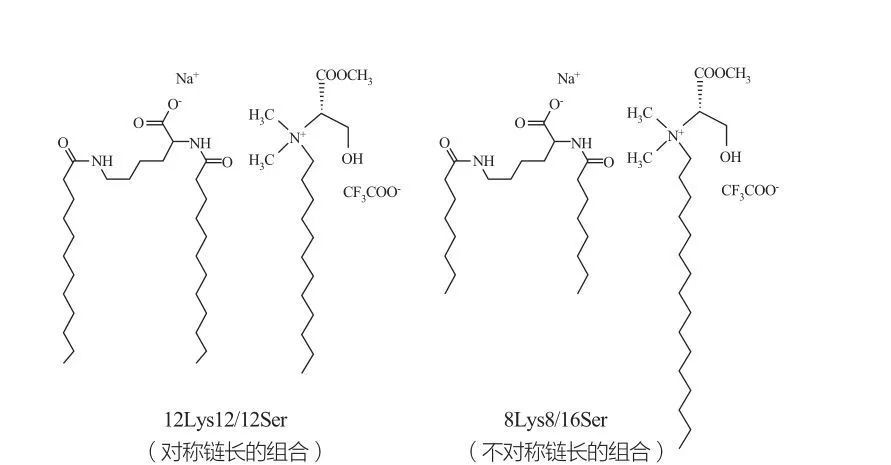
Catanionic mixtures of the lysine- and serine-based surfactants: symmetric 12Lys12/12Ser pair(left) and asymmetric 8Lys8/16Ser pair(right)
6.9 Emulsifying ability
Kouchi et al. examined the emulsifying ability, interfacial tension, dispersibility, and viscosity of N-[3-dodecyl-2-hydroxypropyl]-L-arginine, L-glutamate, and other AAS. In comparison with synthetic surfactants (their conventional nonionic and amphoteric counterparts), the results showed that AAS have stronger emulsifying ability than conventional surfactants.
Baczko et al. synthesized novel anionic amino acid surfactants and investigated their suitability as chiral oriented NMR spectroscopy solvents. A series of sulfonate-based amphiphilic L-Phe or L-Ala derivatives with different hydrophobic tails (pentyl~tetradecyl) were synthesized by reacting amino acids with o-sulfobenzoic anhydride. Wu et al. synthesized sodium salts of N-fatty acyl AAS and investigated their emulsification ability in oil-in-water emulsions, and the results showed that these surfactants performed better with ethyl acetate as the oil phase than with n-hexane as the oil phase.
6.10 Advances in synthesis and production
Hard water resistance can be understood as the ability of surfactants to resist the presence of ions such as calcium and magnesium in hard water, i.e., the ability to avoid precipitation into calcium soaps. Surfactants with high hard water resistance are very useful for detergent formulations and personal care products. Hard water resistance can be evaluated by calculating the change in solubility and surface activity of the surfactant in the presence of calcium ions.
Another way to evaluate hard water resistance is to calculate the percentage or grams of surfactant required for the calcium soap formed from 100 g of sodium oleate to be dispersed in water. In areas with high hard water, high concentrations of calcium and magnesium ions and mineral content can make some practical applications difficult. Often the sodium ion is used as the counter ion of a synthetic anionic surfactant. Since the divalent calcium ion is bound to both surfactant molecules, it causes the surfactant to precipitate more readily from solution making detergency less likely.
The study of the hard water resistance of AAS showed that the acid and hard water resistance were strongly influenced by an additional carboxyl group, and the acid and hard water resistance increased further with the increase of the length of the spacer group between the two carboxyl groups. The order of acid and hard water resistance was C 12 glycinate < C 12 aspartate < C 12 glutamate. Comparing the dicarboxylated amide bond and the dicarboxylated amino surfactant, respectively, it was found that the pH range of the latter was wider and its surface activity increased with the addition of an appropriate amount of acid. The dicarboxylated N-alkyl amino acids showed chelating effect in the presence of calcium ions, and C 12 aspartate formed white gel. c 12 glutamate showed high surface activity at high Ca 2+ concentration and is expected to be used in seawater desalination.
6.11 Dispersibility
Dispersibility refers to the ability of a surfactant to prevent coalescence and sedimentation of the surfactant in solution. Dispersibility is an important property of surfactants that makes them suitable for use in detergents, cosmetics and pharmaceuticals. A dispersing agent must contain an ester, ether, amide or amino bond between the hydrophobic group and the terminal hydrophilic group (or among the straight chain hydrophobic groups).
Generally, anionic surfactants such as alkanolamido sulfates and amphoteric surfactants such as amidosulfobetaine are particularly effective as dispersing agents for calcium soaps.
Many research efforts have determined the dispersibility of AAS, where N-lauroyl lysine was found to be poorly compatible with water and difficult to use for cosmetic formulations. In this series, N-acyl-substituted basic amino acids have superb dispersibility and are used in the cosmetic industry to improve formulations.
07 Toxicity
Conventional surfactants, especially cationic surfactants, are highly toxic to aquatic organisms. Their acute toxicity is due to the phenomenon of adsorption-ion interaction of surfactants at the cell-water interface. Decreasing the cmc of surfactants usually leads to stronger interfacial adsorption of surfactants, which usually results in their elevated acute toxicity. An increase in the length of the hydrophobic chain of surfactants also leads to an increase in surfactant acute toxicity. Most AAS are low or non-toxic to humans and the environment (especially to marine organisms) and are suitable for use as food ingredients, pharmaceuticals and cosmetics. Many researchers have demonstrated that amino acid surfactants are gentle and non-irritating to the skin. Arginine-based surfactants are known to be less toxic than their conventional counterparts.
Brito et al. studied the physicochemical and toxicological properties of amino acid-based amphiphiles and their [derivatives from tyrosine (Tyr), hydroxyproline (Hyp), serine (Ser) and lysine (Lys)] spontaneous formation of cationic vesicles and gave data on their acute toxicity to Daphnia magna (IC 50). They synthesized cationic vesicles of dodecyltrimethylammonium bromide (DTAB)/Lys-derivatives and/or Ser-/Lys-derivative mixtures and tested their ecotoxicity and hemolytic potential, showing that all AAS and their vesicle-containing mixtures were less toxic than the conventional surfactant DTAB.
Rosa et al. investigated the binding (association) of DNA to stable amino acid-based cationic vesicles. Unlike conventional cationic surfactants, which often appear to be toxic, the interaction of cationic amino acid surfactants appears to be non-toxic. The cationic AAS is based on arginine, which spontaneously forms stable vesicles in combination with certain anionic surfactants. Amino acid-based corrosion inhibitors are also reported to be non-toxic. These surfactants are easily synthesized with high purity (up to 99%), low cost, easily biodegradable, and completely soluble in aqueous media. Several studies have shown that sulfur-containing amino acid surfactants are superior in corrosion inhibition.
In a recent study, Perinelli et al. reported a satisfactory toxicological profile of rhamnolipids compared to conventional surfactants. Rhamnolipids are known to act as permeability enhancers. They also reported the effect of rhamnolipids on the epithelial permeability of macromolecular drugs.
08 Antimicrobial activity
The antimicrobial activity of surfactants can be evaluated by the minimum inhibitory concentration. The antimicrobial activity of arginine-based surfactants has been studied in detail. Gram-negative bacteria were found to be more resistant to arginine-based surfactants than Gram-positive bacteria. The antimicrobial activity of surfactants is usually increased by the presence of hydroxyl, cyclopropane or unsaturated bonds within the acyl chains. Castillo et al. showed that the length of the acyl chains and the positive charge determine the HLB value (hydrophilic-lipophilic balance) of the molecule, and these do have an effect on their ability to disrupt membranes. Nα-acylarginine methyl ester is another important class of cationic surfactants with broad-spectrum antimicrobial activity and It is readily biodegradable and has low or no toxicity. Studies on the interaction of Nα-acylarginine methyl ester-based surfactants with 1,2-dipalmitoyl-sn-propyltrioxyl-3-phosphorylcholine and 1,2-ditetradecanoyl-sn-propyltrioxyl-3-phosphorylcholine, model membranes, and with living organisms in the presence or absence of external barriers have shown that this class of surfactants has good antimicrobial The results showed that the surfactants have good antibacterial activity.
09 Rheological properties
The rheological properties of surfactants play a very important role in determining and predicting their applications in different industries, including food, pharmaceuticals, oil extraction, personal care and home care products. Many studies have been conducted to discuss the relationship between viscoelasticity of amino acid surfactants and cmc.
10 Applications in the cosmetic industry
AAS are used in the formulation of many personal care products. potassium N-cocoyl glycinate is found to be gentle on the skin and is used in facial cleansing to remove sludge and makeup. n-Acyl-L-glutamic acid has two carboxyl groups, which makes it more water soluble. Among these AAS, AAS based on C 12 fatty acids are widely used in facial cleansing to remove sludge and makeup. AAS with a C 18 chain are used as emulsifiers in skin care products, and N-Lauryl alanine salts are known to create creamy foams that are not irritating to the skin and can therefore be used in the formulation of baby care products. N-Lauryl-based AAS used in toothpaste have good detergency similar to soap and strong enzyme-inhibiting efficacy.
Over the past few decades, the choice of surfactants for cosmetics, personal care products and pharmaceuticals has focused on low toxicity, mildness, gentleness to the touch and safety. Consumers of these products are acutely aware of the potential irritation, toxicity and environmental factors.
Today, AAS are used to formulate many shampoos, hair dyes and bath soaps due to their many advantages over their traditional counterparts in cosmetics and personal care products. Protein-based surfactants have desirable properties necessary for personal care products. Some AAS have film-forming capabilities, while others have good foaming capabilities.
Amino acids are important naturally occurring moisturizing factors in the stratum corneum. When epidermal cells die, they become part of the stratum corneum and the intracellular proteins are gradually degraded to amino acids. These amino acids are then transported further into the stratum corneum, where they absorb fat or fat-like substances into the epidermal stratum corneum, thereby improving the elasticity of the skin's surface. Approximately 50% of the natural moisturizing factor in the skin is composed of amino acids and pyrrolidone.
Collagen, a common cosmetic ingredient, also contains amino acids that keep the skin soft. Skin problems such as roughness and dullness are due in large part to a lack of amino acids. One study showed that mixing an amino acid with an ointment relieved skin burns, and the affected areas returned to their normal state without becoming keloid scars.
Amino acids have also been found to be very useful in caring for damaged cuticles. Dry, shapeless hair may indicate a decrease in the concentration of amino acids in a severely damaged stratum corneum. Amino acids have the ability to penetrate the cuticle into the hair shaft and absorb moisture from the skin. This ability of amino acid based surfactants makes them very useful in shampoos, hair dyes, hair softeners, hair conditioners, and the presence of amino acids makes the hair strong.
11 Applications in everyday cosmetics
Currently, there is a growing demand for amino acid-based detergent formulations worldwide. AAS are known to have better cleaning ability, foaming ability and fabric softening properties, which makes them suitable for household detergents, shampoos, body washes and other applications. An aspartic acid-derived amphoteric AAS is reported to be a highly effective detergent with chelating properties. The use of detergent ingredients consisting of N-alkyl-β-aminoethoxy acids was found to reduce skin irritation. A liquid detergent formulation consisting of N-cocoyl-β-aminopropionate has been reported to be an effective detergent for oil stains on metal surfaces. An aminocarboxylic acid surfactant, C 14 CHOHCH 2 NHCH 2 COONa, has also been shown to have better detergency and is used for cleaning textiles, carpets, hair, glass, etc. The 2-hydroxy-3-aminopropionic acid-N,N-acetoacetic acid derivative is known to have good complexing ability and thus gives stability to bleaching agents.
The preparation of detergent formulations based on N-(N'-long-chain acyl-β-alanyl)-β-alanine has been reported by Keigo and Tatsuya in their patent for better washing ability and stability, easy foam breaking and good fabric softening. Kao developed a detergent formulation based on N-Acyl-1 -N-hydroxy-β-alanine and reported low skin irritation, high water resistance and high stain removal power.
The Japanese company Ajinomoto uses low-toxic and easily degradable AAS based on L-glutamic acid, L-arginine and L-lysine as the main ingredients in shampoos, detergents and cosmetics (Figure 13). The ability of enzyme additives in detergent formulations to remove protein fouling has also been reported. N-acyl AAS derived from glutamic acid, alanine, methylglycine, serine and aspartic acid have been reported for their use as excellent liquid detergents in aqueous solutions. These surfactants do not increase viscosity at all, even at very low temperatures, and can be easily transferred from the storage vessel of the foaming device to obtain homogeneous foams.
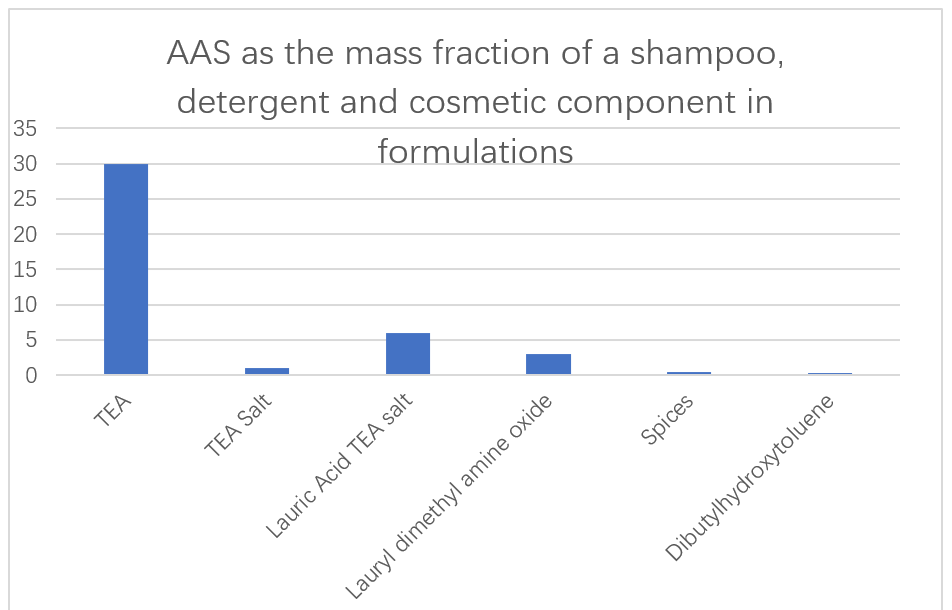
Post time: Jun-09-2022